Phosphates for Battery Applications
Introduction:
Phosphate (and other polyanion-based) lithium-ion batteries represent the next generation of energy
storage devices, combining outstanding electrochemical performance with unrivalled thermal
stability. The use of lithium metal phosphates in lithium-ion battery applications
was first reported by Jerry Barker et al. (US Patent #5871866, filed 1996)
and by J. Goodenough and co-workers (J. Electrochem., Soc., 144, (1997),
1609). These seminal publications demonstrated for the first time the lithium insertion properties of Nasicon
phases (e.g. Li3V2(PO4)3) and olivine based LiMPO4 materials (e.g.
LiFePO4). In addition, the
excellent safety characteristics of these
materials were also defined. Based on these preliminary studies it became
clear that these phosphate materials would form the basis of a new generation of commercially successful lithium-ion batteries.
Background:
The table below briefly summarizes the inventorship and
electrochemical properties of the polyanion materials which are currently
of interest for lithium-ion and sodium-ion applications. Please use the hyperlinks
provided in the table to obtain further information about the issued
patents.
Material |
Nominal
Voltage vs. Li |
Specific
Capacity mAh/g |
Inventor |
Patent# |
Comments |
LiFePO4 |
3.45 |
130-150 |
J. Goodenough |
US5910382 and others |
Olivines |
LiFe1-xMxPO4 |
3.45 |
130-160 |
J.Barker et al. |
US6884544 and others |
M = Mg, Ca, Zn |
Li3V2(PO4)3 |
3.6-4.7 |
197 |
J.Barker et al. |
US5871866 and others |
Nasicons |
LiVPO4F |
4.2 |
155 |
J.Barker et al. |
US6387568 and others |
Triclinic |
LiVPO4.OH |
4.1 |
158 |
J.Barker et al. |
US6777132 |
Triclinic |
LiVP2O7 |
4.1 |
116 |
--- |
--- |
Diphosphates |
Li2MP2O7 |
3.6, 4.2 |
110-220 |
J.Barker
et al. |
US7008566 |
M = Fe, Mn
etc. |
LiMSO4F |
3.5 |
151 |
J.Barker
et al. |
US2005/0163699 |
M = Fe, Mn
etc. |
NaMSO4F |
3.5 |
138 |
J.Barker
et al. |
US2005/0163699 |
M = Fe, Mn
etc. |
Li2MPO4F |
4.7 |
143 |
J.Barker |
US6890686 and others |
M = Co, Ni etc. |
Na2MPO4F |
4.7 |
122 |
J.Barker |
US6890686 and others |
Sodium Ion |
Li4V2(SiO4)(PO4)2 |
3.6-4.7 |
260 |
J.Barker |
US6136472 and others |
Silicophosphates |
Li3V1.5Al0.5(PO4)3 |
3.6-4.7 |
203 |
J.Barker |
US5871866 and others |
Nasicons |
β-LiVOPO4 |
4.0 |
159 |
J.Barker |
US6645452 (CTR) |
Prepared by CTR |
NaVPO4F |
3.7 |
143 |
J.Barker |
US6872492 and others |
Sodium Ion |
Na3V2(PO4)2F3 |
3.7 |
192 |
J.Barker |
US6872492 and others |
Sodium Ion |
Novel Phase A |
3.8 |
ca. 150 |
J.Barker |
Pending |
Application Pending |
Novel Phase B |
3.9 |
ca. 140 |
J.Barker |
Pending |
Application Pending |
Novel Phase C |
3.5 |
ca. 145 |
J.Barker |
Pending |
Application Pending |
Table Notes:
1. A specific example phase is given in each case -
the patent coverage generally extends to all generic materials within the
particular class.
2. The patent numbers quoted are for example only. Please refer
to our Patents webpage for further details on the general patent
coverage.
3. The Novel Polyanion Phases A to C are currently under development
and further details will be provided when the related patent applications
are granted.
4. CTR = Carbothermal Reduction. This is the patented
method used for the economical preparation of these materials. See
below for more details.
5. The NaVPO4F and Na3V2(PO4)2F3 materials may find
application in both Sodium-ion battery applications and in next generation
Hybrid-ion Cells.
6. The specific capacity figures quoted for LiFePO4 and
LiFe1-xMxPO4 are
estimates for this material in a lithium-ion configuration.
Nasicon Phases (e.g. Li3M2(PO4)3):
The original Nasicon related patent (US#5871866), awarded to Jerry
Barker and co-workers described the electrochemical insertion properties
of Li3V2(PO4)3 (LVP) and other iso-structural polyanion phases. In these
preliminary studies on LVP a reversible specific capacity of around 130
mAh/g was revealed, equivalent to the cycling of 2 Li ions per formula
unit. Further investigations by Barker and co-workers and by the
academic groups led by Nazar and Masquelier, later demonstrated that all
three lithium ions could be cycled, equivalent to a remarkable material
utilization of over 190 mAh/g. This specific capacity performance when considered
in conjunction with an average discharge voltage around 4 V vs. Li makes
LVP an excellent cathode active mass for high energy density lithium-ion batteries. Moreover,
DSC studies on charged LVP cathodes also confirmed the outstanding thermal
stability properties of the LVP material.
Recent advances have seen the first introduction of commercial LVP
lithium ion batteries. These devices promise unparalleled rate and safety performance
and may well find application in power tool markets as well as other
consumer applications were high power and good safety are
required.
Olivine Phases (e.g. LiFePO4):
The olivine LiFePO4 phases offers the desirable
combination of low cost, favorable electrochemical activity and low environmental
impact. The LiFePO4 material demonstrates reversible lithium insertion at
around 3.4 V vs. Li, so when combined with graphite in a lithium-ion configuration
generates a cells voltage around 3.2 V.
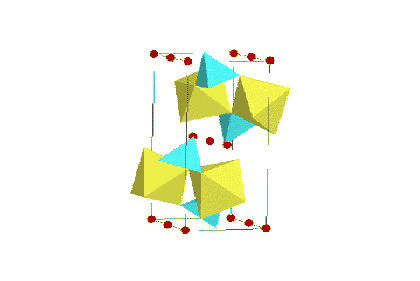
A schematic representation of the LiFePO4 olivine
structure
The LiFePO4 material is normally prepared using relatively
high cost Fe2+ precursors. Recent investigations have led to improvements
in the material utilization of the LiFePO4 by the addition of a conductive
carbon layer, although this leads to a even more expensive synthesis approach.
Pioneering work by Jerry Barker and co-workers has led to the use of the carbothermal reduction method, which allows a single step synthesis using
low cost precursors such as Fe2O3.
Triclinic Fluorophosphate Phases (e.g. LiVPO4F):
For most lithium-ion applications, LiVPO4F may represent
the ‘jewel in the crown’ of phosphate based active materials. As we
have previously discussed, there is considerable current interest in the
use of phosphate-based active phases such as LiFePO4 (Olivine) and
Li3V2(PO4)3 (Nasicon). In an
important extension of this polyanion approach,
recent studies by Jerry Barker and his co-workers have focused on a series
of novel transition metal fluorophosphate phases, LiMPO4F (where M
represents a 3d transition
metal). This work by Barker et al. has culminated in a number of high
profile publications and issued US patents (for more details please refer
to our Patents and Publications webpages). In particular, the
lithium vanadium fluorophosphate, LiVPO4F phase offers considerable
promise as a viable cathode material for commercial lithium-ion batteries.
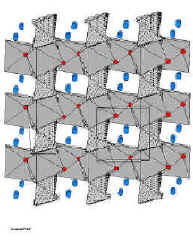
A schematic representation of the LiVPO4F Structure
The LiVPO4F is iso-structural with the
known mineral Ambygonite, LiAlPO4F, crystallizing with a triclinic
structure (space group, P-1). Performance
evaluation of full graphite//LiVPO4F lithium-ion cells indicates a
reversible material utilization for the LiVPO4F of around 130 mAh/g and an
average discharge voltage of 4.06 V.
Long term cycling (C/5 rate at 23 C) of this lithium-ion
configuration predicts a cycle life > 500
cycles to around 90 % of the original discharge capacity – truly
outstanding performance. By comparison with lithium-ion cells based
on the established material LiCoO2, the fluorophosphate material offers a
superior voltage profile, a higher average discharge voltage and
substantially improved safety characteristics. The material is also fully
compatible with existing lithium-ion cell ‘infra-structure’ such as
current electrolyte formulations and charge circuit electronics.
Other Phosphate Materials:
Among the other polyanion active materials developed by
Jerry Barker are LiVOPO4, Na3V2(PO4)2F3, LiVP2O7 and NaVPO4F. These phases
have already found application in, for example, sodium-ion applications
and hybrid-ion cells.
Carbothermal Reduction:
Carbothermal
Reduction (CTR) was invented and patented by Jerry Barker and his
co-workers as an economical synthesis method for the large scale
production of polyanion and oxide active materials. In a series of
publications the CTR approach has been described by Barker et al.
as a viable and scalable method to prepare electroactive materials such as
g-LiV2O5
and Li3V2(PO4)3 , LiFePO4, LiVOPO4, LiVPO4F and others.
Valence Technology Inc. has been using
CTR for more than 4 years to make an Olivine and Nasicon active materials
at a commercial scale.
So what was the
problem to be solved? In previous studies the synthesis of LiFePO4 and
other phosphate active materials had often involved the application of
relatively expensive precursors and routinely involved the use of
multi-step reactions. Overall these preparative approaches were not
considered economically viable. What the industry needed was a low cost, single step
preparative approach that yielded a high purity product. In addition, due
to the relatively poor electronic conductivity of most phosphate active
materials, a desirable material would comprise a composite product formed
from the active phase intermingled with finely dispersed carbon.
This is where CTR comes in.
The underlying CTR process is used extensively in the
extraction metallurgy industry to reduce metal oxides (and other
compounds) to the pure metal state (e.g. in the iron and steel blast
furnace) and relies on the application of the two carbon oxidation
reactions:
C
+ O2
®
CO2 (1)
2
C +
O2
®
2 CO
(2)
In essence, the CTR method is a solid-state approach that utilizes
particulate carbon as a selective reducing agent. Advantageously, the
high-surface area carbon acts as convenient nucleation sites for
material growth as well as aiding material conductivity.
|